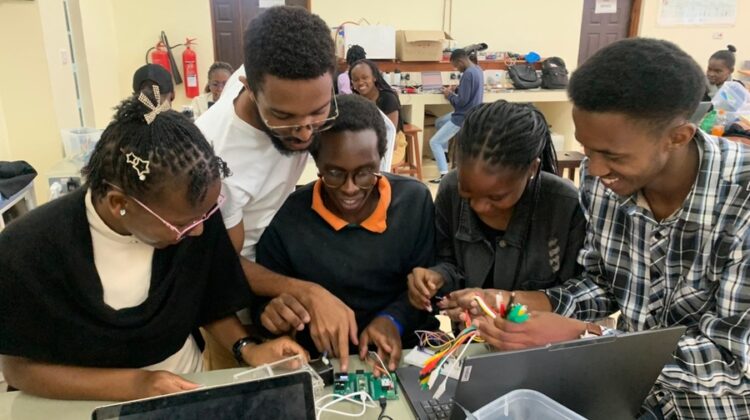
Through workshops based on a course, a team of researchers at MIT has developed a ‘biomedical lab in a box’ that they’ve used to give students in Kenya and Uganda hands-on experience engineering medical hardware.
Globally, and especially in low- and middle-income countries (LMICs), a significant portion of the population lacks access to essential health-care services. Although there are many contributing factors that create barriers to access, in many LMICs failing or obsolete equipment plays a significant role.
‘Those of us who have investigated health-care systems in LMICs are familiar with so-called “equipment graveyards”,’ said Nevan Hanumara, a research scientist in MIT’s Department of Mechanical Engineering, describing piles of broken, imported equipment, often bearing stickers indicating their origins from donor organisations. ‘Looking at the root causes of medical equipment failing and falling out of service in LMICs, we find that the local biomedical engineers truly can’t do the maintenance, due to a cascade of challenges.’
Among these challenges are: design weaknesses – systems designed for temperate, air-conditioned hospitals and stabilised power don’t fare well in areas with inconsistent power supply, dust, high heat and humidity, and continuous utilisation; lack of supply chain – parts ordered in the USA can arrive in days, where parts ordered to East Africa may take months; and limited access to knowledgeable professionals – outside of major metropolitan areas, biomedical engineers are scarce.
Hanumara, Leroy Sibanda, a recent graduate with a dual degree in management and electrical engineering and computer science (EECS), and Anthony Pennes, a technical instructor in EECS, began to ponder what could be changed if local biomedical engineers were actually involved with the design of the equipment that they’re charged with maintaining.
Pennes, who staffs a class on Medical Device Design, among other courses, developed hands-on biosensing and mechatronics exercises as class activities several years ago. Hanumara became interested in expanding that curriculum to produce something that could have a larger impact.
Working as a team, and with support from MIT International Science and Technology Initiatives (MISTI), the MIT Jameel World Education Lab and the Priscilla King Gray Public Service Center, the trio created a hands-on course, exercises and curriculum, supported by what they’ve now dubbed a ‘biomed lab in a box’ kit.

Sibanda, who hails from Bulawayo, Zimbabwe, brought additional lived experience to the project. He said friends up and down the continent speak about great practical primary and secondary education, and a tertiary education that provides a heavy emphasis on theory. The consequence, he said, is a plethora of graduates who are absolutely brilliant at the theory, but less experienced in advanced practical concepts.
‘Anyone who has ever had to build systems that need to stand up to real-world conditions understands the chasm between knowing how to calculate the theoretically perfect “x” and being capable of implementing a real-world solution with the materials available,’ said Sibanda.
Hanumara and Sibanda travelled to Nairobi, Kenya, and Mbarara, Uganda, in late 2024 to test their kit and their theory, teaching three-day long biomedical innovation mini-courses at both Kenyatta University and Mbarara University of Science and Technology (MUST), with Pennes providing remote support from MIT’s campus.
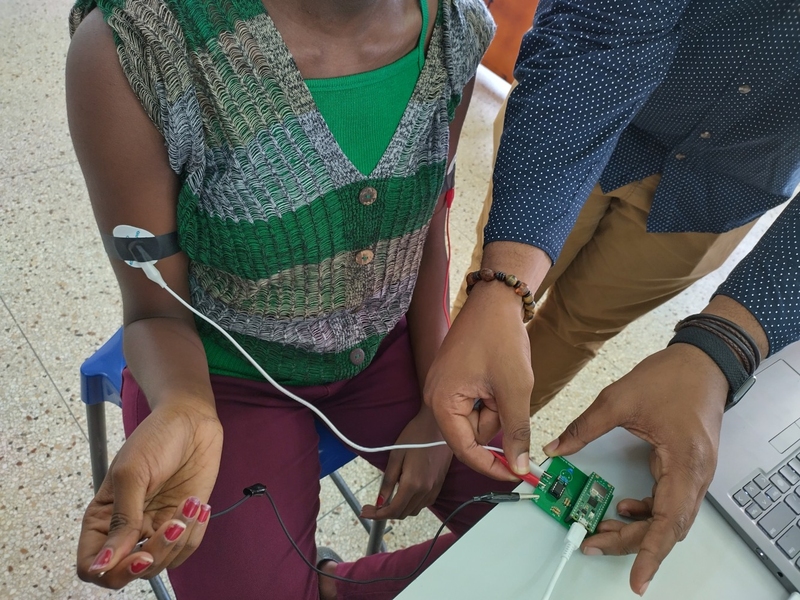
The mini-courses were designed to connect the theoretical to the physical, increasing in complexity and confronting students with the real challenges of biomedical hardware and sensing, such as weak signals, ambient noise, motion artifacts, debugging and precision assembly.
Pennes said the goal for the mini-courses was to shape the project around the real-world experiences of the region’s biomedical engineering students. ‘One of the problems that they experience in this region is not simply a lack of equipment, but the lack of ability to maintain it,’ he said. ‘Some organisation will come in and donate thousands of dollars of surgical lighting; then a power supply will burn out, and the organisation will never come back to fix it.’
But that’s just the beginning of the problem, he adds. Engineers often find that the design isn’t open and there’s no manual, making it impossible to find a circuit design for what’s inside the donated, proprietary system. ‘You have to poke and prod around the disassembled gear to see if you can discern the makers’ original goals in wiring it and figure out a fix,’ said Pennes.
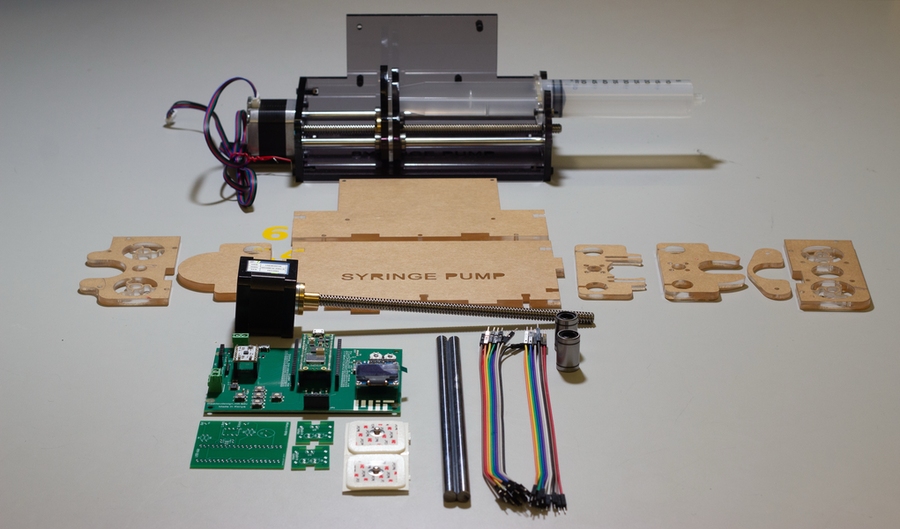
In one example, he recalls seeing a donated screen for viewing X-rays – the lightbox kind, used to backlight film so that technicians can read the image – with a burned-out bulb. ‘The screen is lit by a proprietary bulb, so when it burned out, they could not replace it,’ he said.
Local biomedical engineers ultimately realised that they could take a number of off-the-shelf fluorescent bulbs and angle them to fit inside the box. ‘Then they sort of MacGyver’d the wiring to make them all work. You get the medical technology to work however you can.’
It’s this hands-on, imaginative approach to problem-solving that the team hopes to promote. Each of the course modules is designed to be a self-contained learning experience, and the kit is accompanied by a USB flash drive with a 96-page lab manual written by Sibanda, and all the needed software, which is important to have when internet access is unreliable.
‘Our mission was to expose eager, young biomedical engineers to the hands-on, ‘mens-et-manus’ (‘mind-and-hand’) culture that is the cornerstone of MIT, and encourage them to develop their talents and aspirations as engineers and innovators,’ said Hanumara. ‘We wanted to help empower them to participate in developing high-quality, contextually appropriate, technologies that improve health-care delivery in their own region.’