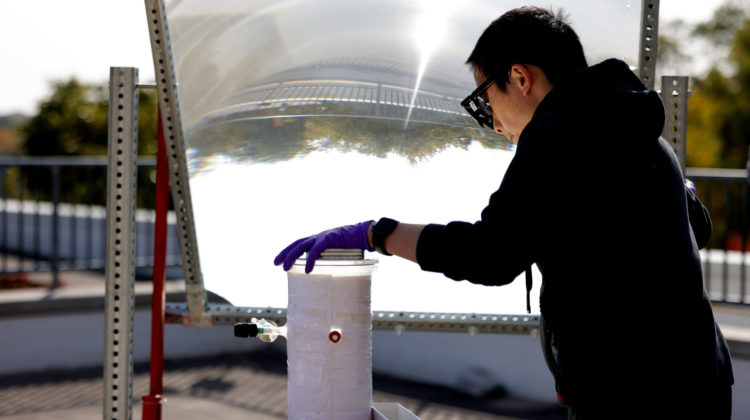
A new type of solar panel developed at the University of Michigan has achieved nine per cent efficiency in converting water into hydrogen and oxygen – mimicking a crucial step in natural photosynthesis. Outdoors, it represents a major leap in the technology, nearly ten times more efficient than similar solar water-splitting experiments.
However, the new panel’s biggest benefit is its reduction in the cost of sustainable hydrogen, the result of shrinking the semiconductor, typically the most expensive part of such a device. The team’s self-healing semiconductor can withstand concentrated light equivalent to that of 160 suns.
Currently, hydrogen is produced from methane, a process that requires a significant amount of energy. However, plants harvest hydrogen atoms from water using sunlight. As humanity tries to reduce its carbon emissions, hydrogen is attractive as both a standalone fuel and as a component in sustainable fuels made with recycled carbon dioxide. Likewise, it’s needed for many chemical processes, such as the production of fertilisers.
‘In the end, we believe that artificial photosynthesis devices will be much more efficient than natural photosynthesis, which will provide a path toward carbon neutrality,’ said Zetian Mi, a professor of electrical and computer engineering.
The new panel is the result of two advances. The first is the ability to concentrate the sunlight without destroying the semiconductor that harnesses the light.
‘We reduced the size of the semiconductor by more than 100 times compared to some semiconductors only working at low light intensity,’ said Peng Zhou, U-M research fellow in electrical and computer engineering. ‘Hydrogen produced by our technology could be very cheap.’
The second advance involved the use of the higher energy part of the solar spectrum to split water and the lower part of the spectrum to provide heat that encourages the reaction. This was achieved by using a semiconductor catalyst that improves itself with use, resisting the degradation that such catalysts usually experience when they harness sunlight to drive chemical reactions.
In addition to handling high light intensities, the semiconductor can thrive in high temperatures that would be punishing to computer semiconductors. Higher temperatures speed up the water-splitting process and the extra heat also encourages the hydrogen and oxygen to remain separate, rather than renewing their bonds and forming water once more. Both of these phenomena helped the team to harvest more hydrogen.
For the outdoor experiment, Zhou set up a lens about the size of a house window to focus sunlight onto an experimental panel just a few centimetres across. Within that panel, the semiconductor catalyst was covered in a layer of water that bubbled with the hydrogen and oxygen gasses that it separated.
The catalyst is made of indium gallium nitride nanostructures grown onto a silicon surface. That semiconductor wafer captures the light, converting it into free electrons and holes – positively charged gaps left behind when electrons are liberated by the light. The nanostructures are peppered with nanoscale balls of metal, 1/2000th of a millimetre across, that use those electrons and holes to help direct the reaction.
A simple insulating layer atop the panel keeps the temperature at 75°C – warm enough to help encourage the reaction while also being cool enough for the semiconductor catalyst to perform well. The outdoor version of the experiment, with less reliable sunlight and temperature, achieved 6.1 per cent efficiency at turning the energy from the sun into hydrogen fuel; indoors, the system achieved nine per cent efficiency.
The next challenges the team intends to tackle are to further improve the efficiency and to achieve ultra-high-purity hydrogen that can be directly fed into fuel cells.
The research has been published in Nature.