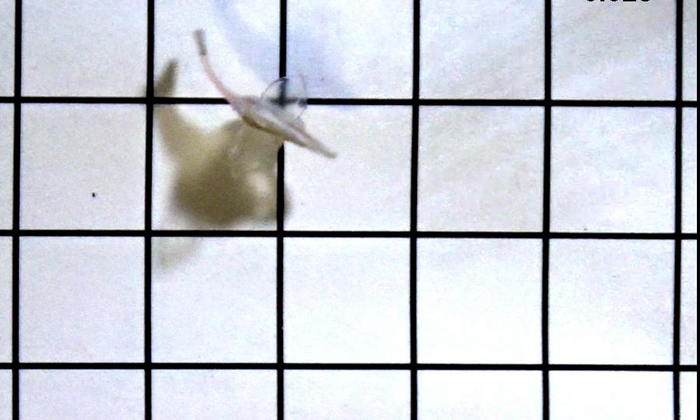
A team of engineers from Harvard and Emory universities have developed the first fully autonomous biohybrid fish made from human-stem-cell-derived cardiac muscle cells. The artificial fish, which swims by recreating the muscle contractions of a pumping heart, represents a big step towards the development of an artificial muscular pump that could be used to study heart diseases such as arrhythmia.
‘Our ultimate goal is to build an artificial heart to replace a malformed heart in a child,’ said Kit Parker, the Tarr Family professor of bioengineering and applied physics at the Harvard John A Paulson School of Engineering and Applied Sciences (SEAS). ‘Most of the work in building heart tissue or hearts, including some work we have done, is focused on replicating the anatomical features or replicating the simple beating of the heart in the engineered tissues. But here, we are drawing design inspiration from the biophysics of the heart, which is harder to do. Now, rather than using heart imaging as a blueprint, we are identifying the key biophysical principles that make the heart work, using them as design criteria, and replicating them in a system, a living, swimming fish, where it is much easier to see if we are successful.’
The fish, which represents the first autonomous biohybrid device made from human-stem-cell-derived cardiomyocytes, was inspired by the shape and swimming motion of a zebrafish. It features two layers of muscle cells, one on each side of the tail fin. When one side contracts, the other stretches, triggering the opening of a mechano-sensitive protein channel. This causes the muscle cells to contract, which triggers a stretch, leading to a closed loop system that can propel the fish for more than 100 days.
‘By leveraging cardiac mechano-electrical signalling between two layers of muscle, we recreated the cycle where each contraction results automatically as a response to the stretching on the opposite side,’ said Keel Yong Lee, a postdoctoral fellow at SEAS. ‘The results highlight the role of feedback mechanisms in muscular pumps such as the heart.’
The researchers also engineered an autonomous pacing node, similar to a pacemaker, which controls the frequency and rhythm of the spontaneous contractions. Together, the two layers of muscle and the autonomous pacing node enabled the generation of continuous, spontaneous and coordinated, back-and-forth fin movements.
‘Because of the two internal pacing mechanisms, our fish can live longer, move faster and swim more efficiently than previous work,’ said Sung-Jin Park, a former postdoctoral fellow in the Disease Biophysics Group at SEAS. ‘This new research provides a model to investigate mechano-electrical signalling as a therapeutic target of heart rhythm management and for understanding pathophysiology in sinoatrial node dysfunctions and cardiac arrhythmia.’
Like a normal animal muscle, the biohybrid fish’s performance improved with exercise. The amplitude of its muscle contractions, maximum swimming speed and muscle coordination all increased for the first month as the cardiomyocyte cells matured. Eventually, the biohybrid fish reached speeds and swimming efficacy similar to those of wild zebrafish.
The team now aims to build even more complex biohybrid devices using human heart cells.
‘I could build a model heart out of Play-Doh, but it doesn’t mean I can build a heart,’ said Parker. ‘You can grow some random tumour cells in a dish until they curdle into a throbbing lump and call it a cardiac organoid. Neither of those efforts is going to, by design, recapitulate the physics of a system that beats more than a billion times during your lifetime while simultaneously rebuilding its cells on the fly. That is the challenge. That is where we go to work.’
The research has been published in Science.