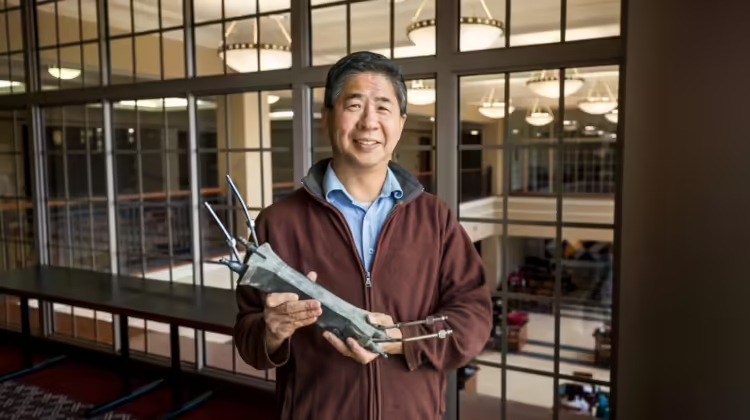
A team of University of Wisconsin-Madison engineers has combined topology optimisation and additive manufacturing to create a twisty high-temperature heat exchanger that outperformed a traditional straight channel design in heat transfer, power density and effectiveness. They used an innovative technique to 3D print – and test – the metal proof of concept.
High-temperature heat exchangers are essential components in many technologies for dissipating heat, with applications in aerospace, power generation, industrial processes and aviation.
‘Traditionally, heat exchangers flow hot fluid and cold fluid through straight pipes, mainly because straight pipes are easy to manufacture,’ said Xiaoping Qian, a professor of mechanical engineering at UW-Madison. ‘But straight pipes are not necessarily the best geometry for transferring heat between hot and cold fluids.’
Additive manufacturing enables researchers to create structures with complex geometries that can yield more efficient heat exchangers. Given this design freedom, Qian set out to discover a design for the hot and cold fluid channels inside a heat exchanger that would maximise heat transfer.
He harnessed his expertise in topology optimisation, a computational design approach used to study the distribution of materials in a structure to achieve certain design goals. He also incorporated a patented technique, called projected undercut perimeter, that considers manufacturability constraints for the overall design.
With an optimised design in hand, Qian worked with colleague Dan Thoma, a professor of materials science and engineering at UW-Madison, who led the 3D printing of the heat exchanger using a metal additive manufacturing technique called laser powder bed fusion.
From the outside, the optimised heat exchanger looks identical to a traditional version with a straight channel design, but their internal core designs are strikingly different. The optimised design has intertwining hot and cold fluid channels with intricate geometries and complex surface features. These complex geometric features guide fluid flow in a twisting path that enhances the heat transfer.
Collaborator Mark Anderson, a professor of mechanical engineering at UW-Madison, conducted thermal-hydraulic tests on the optimised heat exchanger and a traditional heat exchanger to compare their performance. The optimised design was not only more effective in transferring heat, but also achieved a 27 per cent higher power density than the traditional heat exchanger. That higher power density enables a heat exchanger to be lighter and more compact –useful attributes for aerospace and aviation applications.
While previous research has used topology optimisation to study two-fluid heat exchanger designs, Qian said this work is the first to harness topology optimisation and impose manufacturability constraints to ensure the design can be built and tested.
‘Optimising design on the computer is one thing, but to actually make and test it is a very different thing,’ Qian said. ‘It’s exciting that our optimisation method worked. We were able to actually manufacture our heat exchanger design. And, through experimental testing, we demonstrated the performance enhancement of our optimised design. The excellent work performed by the students, postdoctoral researchers and scientists in the three research groups made this advance possible.’
The research has been published in International Journal of Heat and Mass Transfer.